Introduction
Contact tracing has been recognized as a critical process in controlling infectious disease epidemics. Its objectives include early identification of potentially infectious cases and prevention of the emergence of new clusters (1). In the context of the severe acute respiratory syndrome coronavirus 2 (SARS-CoV-2) infection, in-hospital contact-tracing efforts ensure the well-being of frontline healthcare workers (HCWs) to maintain essential healthcare services (2). For this reason, many hospitals from different nations implemented infection risk assessments for HCWs according to international/national guidelines, institutional needs, and resource capacities (3-6). Yet, the key measures to evaluate infection risk were based on exposure: use of masks, contact distance and duration (7, 8).
Large-scale hospital-based studies found household exposure (3, 9), contact with a patient (3), being symptomatic (5), and inadequate use of personal protective equipment (PPE) (9) as the significant factors that contributed to testing positive for SARS-CoV-2 after exposure. In addition, three studies in Türkiye found that high-risk exposures (<1-meter distance, >15-minute duration, no mask) had a higher risk of infection (10-12). The work environment and contact type also affected exposure and SARS-CoV-2 infection risk (12); for example, the index case was more likely to be another coworker (11, 13).
Early on in the SARS-CoV-2 pandemic, international organizations proposed infection risk assessment algorithms for HCWs. The World Health Organization (WHO) recommended infection risk assessment using only the exposure risk. The WHO classified exposure risk as low and high based on contact characteristics (i.e., use of masks, contact distance, and duration). However, the complexity of in-hospital contact scenarios and not including symptom assessment in the algorithms resulted in risk categorization and management limitations. Moreover, contact tracing and exposure risk assessment were time-consuming and resource-intensive processes requiring trained personnel to ensure standardized evaluation (14, 15).
Given these challenges, a need for an accurate and easy-to-use contact tracing strategy for respiratory virus infections that require a rapid, global response in the future remains. This study aimed to evaluate models derived from real-world hospital-based contact tracing data to predict SARS-CoV-2 infection in a simplified manner.
Materials and Methods
In this retrospective cohort study, we examined all SARS-CoV-2 infection and contact tracing data collected between March 23, 2020, and October 22, 2021, from HCWs at the Dokuz Eylül University Research and Application Hospital in İzmir, Türkiye. The hospital had a workforce of nearly 4000 employees, and during the pandemic, provided both outpatient and inpatient care for coronavirus disease 2019 (COVID-19) patients.
Dokuz Eylül University Non-Interventional Research Ethics Committee approved the study with the number 2022/17-15.
Risk Assessment Procedure
Upon identifying a confirmed COVID-19 case among HCWs, our institution implemented rigorous contact tracing and risk assessment procedures. If the primary case was an HCW, a list of potentially exposed coworkers was compiled for contact tracing, and the contact tracing period started two days before symptom onset for symptomatic cases. For asymptomatic cases, contact tracing started two days before the first positive SARS-CoV-2 RT-PCR (RT-PCR) test result. All coworkers potentially exposed to an infected case during these periods were identified. Trained public health residents conducted telephone interviews with the exposed HCWs under the supervision of senior occupational health and epidemiology professionals. Symptoms associated with the illness were evaluated as fever, cough, respiratory symptoms, myalgia and malaise, loss of taste and smell, and diarrhea.
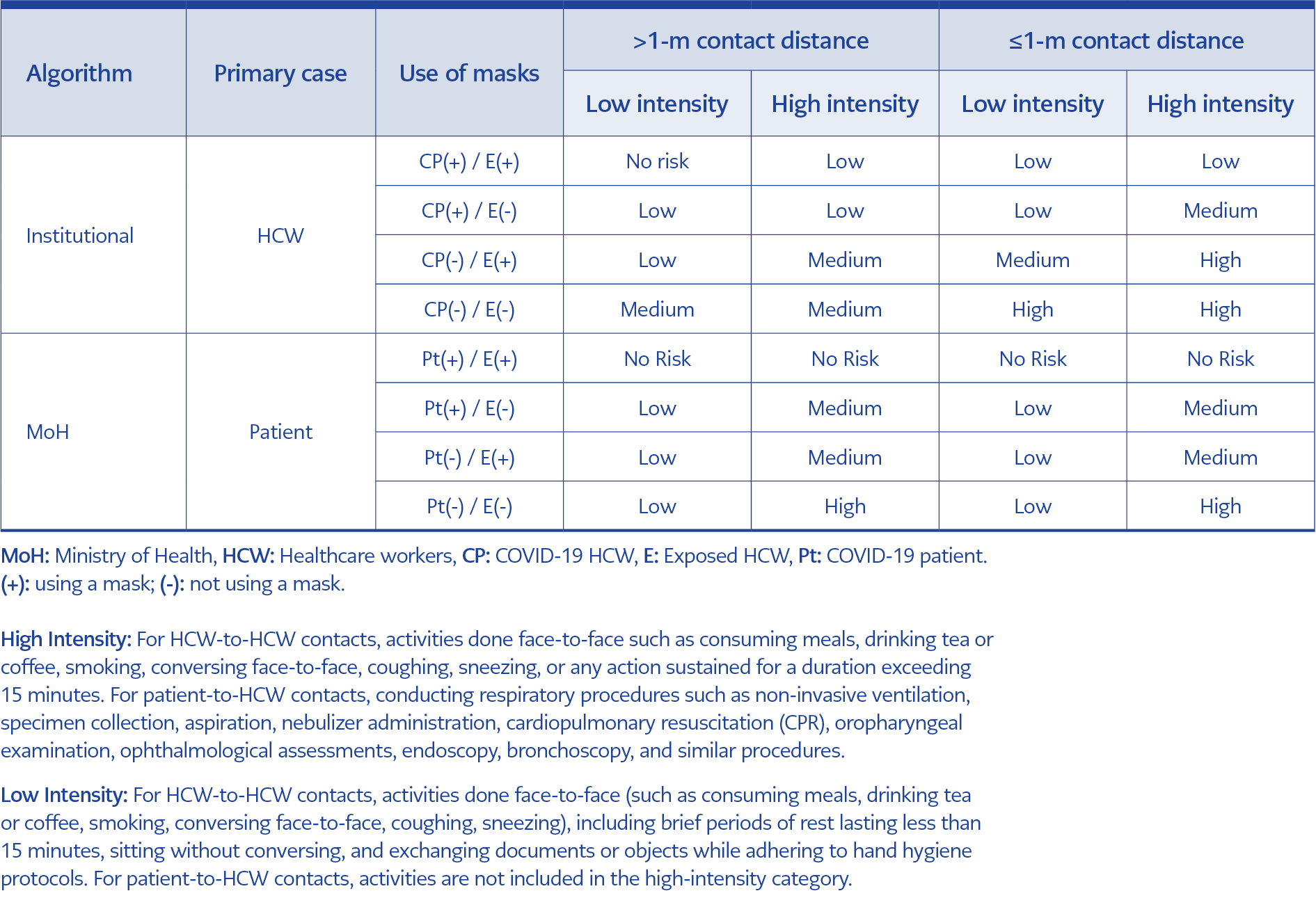
Table 1. Levels of exposure risk for HCWs: algorithms from institutional and Ministry of Health sources based on the primary case, use of masks, contact distance and intensity.
We utilized exposure risk assessment using two distinct algorithms: one for patient-to-HCW contacts and the other for HCW-to-HCW contacts. Patient-to-HCW contacts were assessed following the guidelines of the Turkish Ministry of Health (16). This national algorithm included the patient’s mask use, the HCW’s mask use, and PPE suitability for healthcare procedures. Since no national guidelines were available for evaluating contacts among HCWs, our institution developed an algorithm for HCW-to-HCW interactions, which was aligned with international guidelines and expert opinions within our institution. The risk assessment algorithm for HCW-to-HCW contacts included contact duration, distance, mask use, and the intensity of interactions, such as handshaking or having meals or coffee together (13). Both algorithms are summarized in Table 1.
Following the assessment, exposure risk levels were classified as low, medium, or high. Low or medium-risk HCWs were permitted to continue working while being monitored for symptoms for 14 days from the last contact with a SARS-CoV-2 infected person. Medium-risk HCWs underwent an RT-PCR test on the seventh day of the first contact. High-risk HCWs were subject to work restrictions and home quarantined until RT-PCR testing was conducted on the seventh day. After the assessment, informative text messages detailing work restrictions, quarantine procedures, and scheduled RT-PCR testing dates were sent to the HCWs. Routine RT-PCR testing was recommended for medium to high-risk HCWs but not for low-risk HCWs. Regardless of risk category, HCWs experiencing any symptoms were instructed to immediately report to the occupational health outpatient clinic for the same-day RT-PCR testing.
Study Variables
Professions were categorized as physicians (medical doctors, dentists, pharmacists), nurses, allied healthcare personnel (laboratory technicians, dieticians, social workers, physiotherapists, anesthetic technicians), auxiliary staff (administrative staff, secretaries, office workers), and support staff (cleaning staff, porters, repair workers, garden maintenance staff, kitchen workers, security staff). Symptoms identified in the risk assessment phone interview were acknowledged as symptom presence. HCWs vaccinated with at least two doses of inactivated or mRNA COVID-19 vaccines 14 days before the exposure was classified as two-dose vaccinated. A positive RT-PCR result at least 14 days before the first exposure date was defined as a previous infection. The use of respiratory or surgical masks constituted PPE use. We followed the exposed workers until 14 days after the last contact with a SARS-CoV-2-infected person and assessed their RT-PCR test results within that timeframe. Individuals with all negative results were considered PCR-negative, and those with at least one positive result were regarded as PCR-positive, regardless of any negative results.
Statistical Analysis
Categorical variables were presented as numbers with percentages (n, %) and were analyzed using the chi-squared test. According to the normal distribution assessment, the Student’s t-test or the Mann-Whitney U test was conducted to compare continuous variables. Factors associated with reporting a positive PCR test were evaluated using logistic regression among the contacted HCWs who had RT-PCR testing. The measure of risk was the odds ratio (OR) with 95% confidence interval (CI).
To investigate the practical use of contact and risk assessment-based models incorporating symptoms and other variables, we built four different models with the “enter” method. Model 1 and Model 2 were contact-based models. Model 1 involved an assessment of the globally accepted measures: use of PPE, contact duration, and distance. Model 2 incorporated Model 1 variables plus the presence of symptoms. For Model 3, we built a risk assessment model utilizing the risk categories (low, medium, and high risk) as summarized in Table 1. In Model 4, we included Model 3 variables plus the presence of symptoms. All models were adjusted to account for demographic variables (age, gender), occupational variables (profession, case type), and COVID-19 history (previous infection, vaccination). To compare the discriminative power and fits of the models, we calculated the AUC (area under the curve), Tjur’s R2, and Akaike’s information criterion (AIC). To evaluate the diagnostic accuracy, we computed the diagnostic odds ratio (DOR) for various thresholds of the models, ranging from 1% to 15%. The DOR was calculated as (Sensitivity / [1- Sensitivity]) / ([1-Specificity] / Specificity). The DOR remains unaffected by the prevalence of the disease (17). It quantifies the odds of positive test results in individuals with RT-PCR positivity compared to the odds of positive test results in those without RT-PCR positivity.
As a sensitivity analysis, we repeated all models with inverse probability weighting (IPW) to address potential selection bias stemming from unequal probabilities of RT-PCR testing among risk categories. The variables (age, having symptoms, risk category, and case type) used for IPW were profession and the statistically significant variables in the comparison of RT-PCR-tested and not-tested workers (Supplementary Table 1). Statistical analyses were conducted using R version 4.2.3, with double-sided p-values of less than 0.05 considered significant.
Results
A total of 3389 contacts that occurred from March 23, 2020, to October 22, 2021, were included in the study. The characteristics of exposed HCWs are presented in Table 2. The majority of contacts occurred among colleagues (67.6%). The mean age of the exposed HCWs was 35.2±9.0, and the majority were women (58.7%). Of the contacts, 69.4% occurred within 1 meter of distance, and 60.8% lasted 15 minutes or more. PPE was used in 56.9% of the contacts. Exposure risk was reported as low in 35.0% of the contacts, followed by medium (34.6%) and high risk (30.4%). Of the contacts, 24.3% were experiencing symptoms at the time of risk assessment. Among the 2451 (72.3%) exposed HCWs who had SARS-CoV-2 PCR testing, 144 (5.9%) had a positive RT-PCR test result. Clinically, all cases were asymptomatic or with mild illness with no intensive care unit (ICU) admission or death.
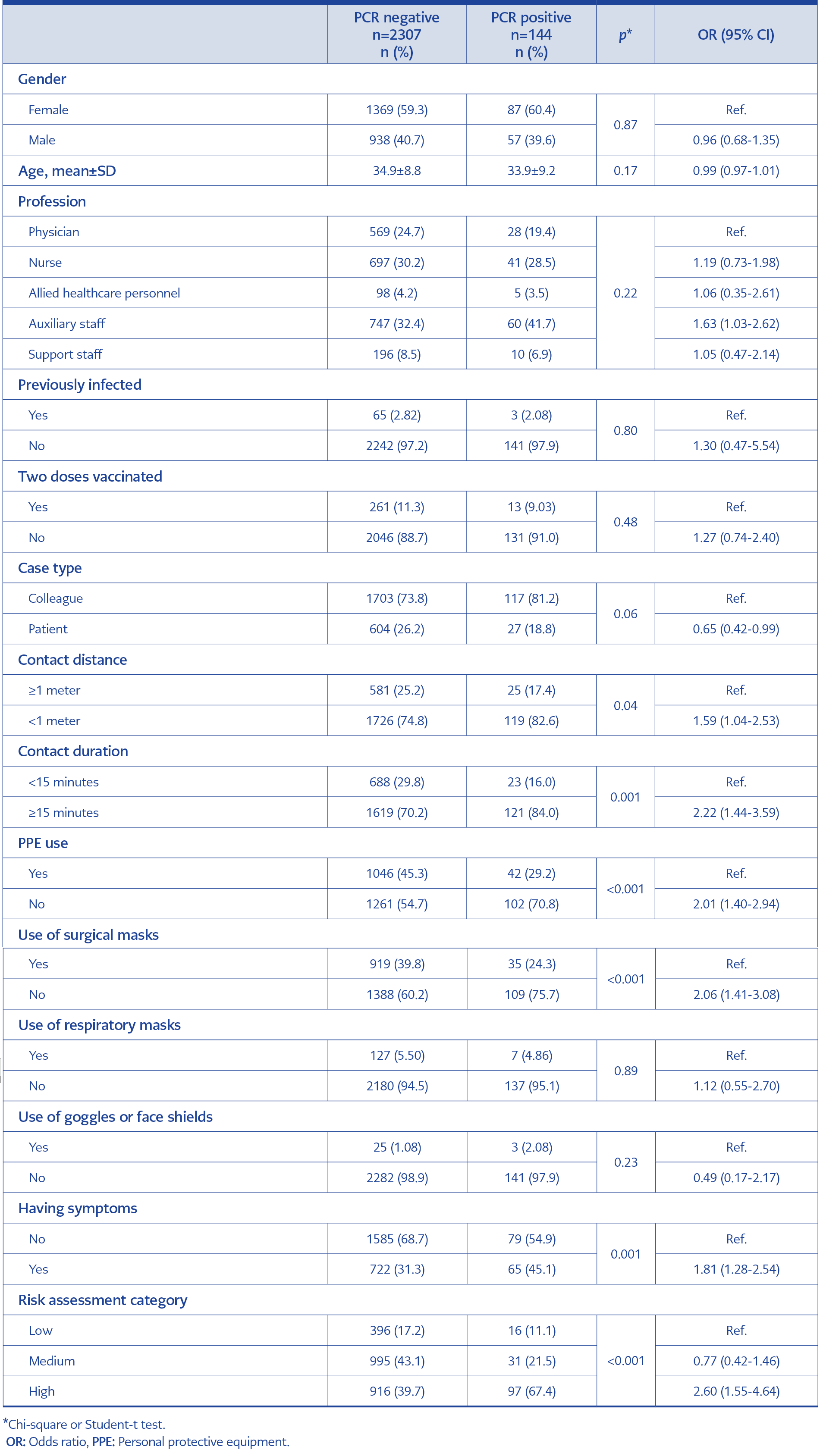
Table 3. Comparison of the HCWs who tested RT-PCR negative and positive within 14 days after the last exposure (n=2451).
A comparison of HCWs who tested positive and negative for PCR after exposure is shown in Table 3. Auxiliary staff showed an increased risk of testing positive compared to physicians, with an OR of 1.63 (95% CI=1.03-2.62). Previously infected (p=0.80) and fully vaccinated individuals (p=0.48) demonstrated no significant difference in PCR positivity within 14 days post-exposure. Contact of < 1-meter (OR=1.59, 95% CI=1.04-2.53), ≥15 minutes duration (OR=2.22, 95% CI=1.44-3.59), no use of PPE (OR=2.01, 95% CI=1.40-2.94), and having symptoms (OR=1.81, 95% CI=1.28-2.54) were associated with a significant increase in the odds of PCR positivity.
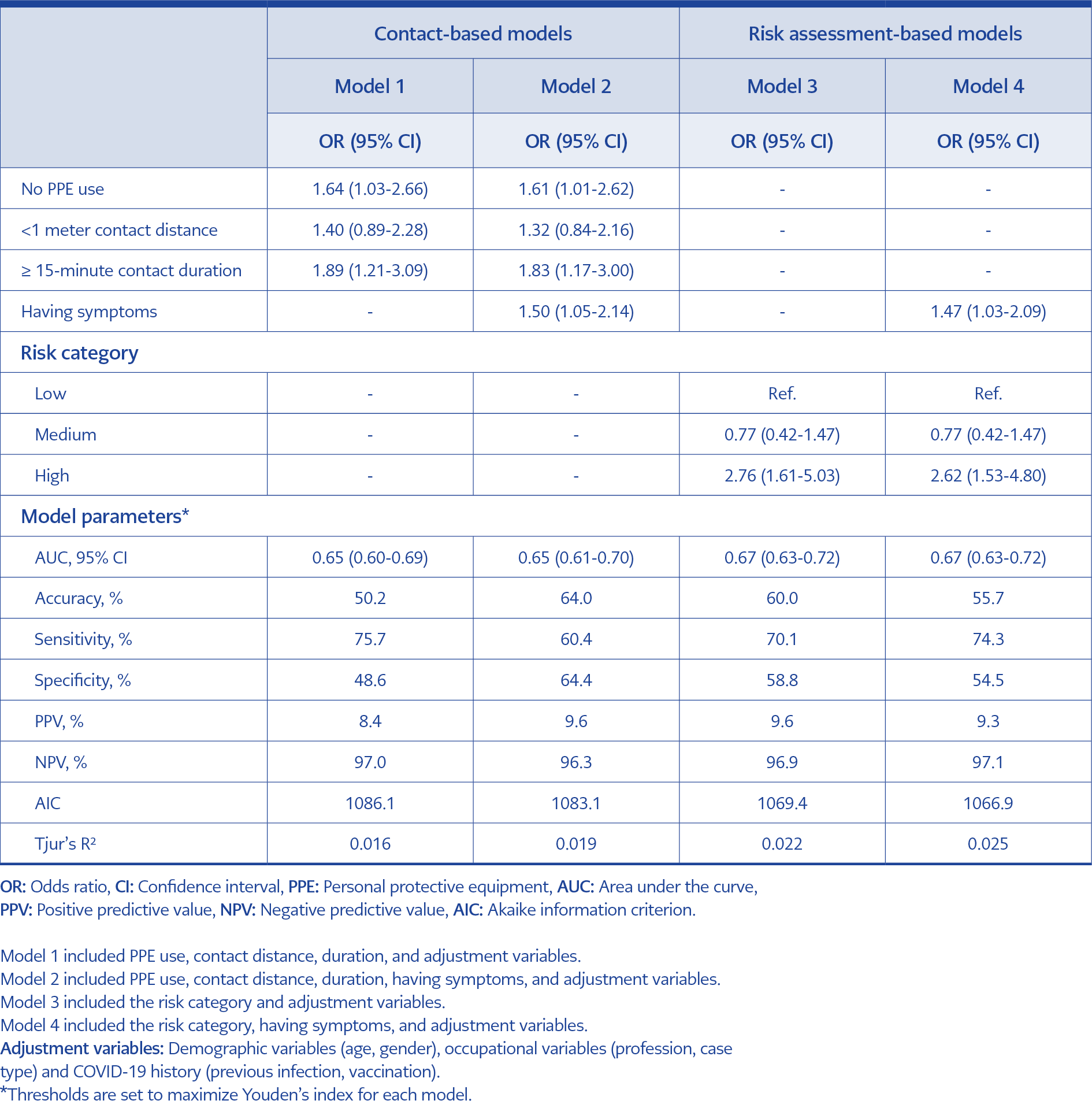
Table 4. Logistic regression results using contact-based and risk assessment-based models predicting SARS-CoV-2 RT-PCR positivity within 14-days after the last exposure.
Table 4 presents logistic regression results for the PCR positivity within 14 days after the last exposure. In the contact-based Model 1, no PPE use (OR=1.64, 95% CI=1.03-2.66) and ≥15 minutes of contact duration (OR=1.89, 95% CI=1.21-3.09) were significantly associated with PCR positivity. In Model 2, symptoms were added to Model 1 variables, which improved model parameters (AIC from 1086.1 to 1083.1; Tjur’s R2 from 0.016 to 0.019, respectively). According to the risk assessment-based Model 3, being evaluated as a high-risk contact increased the odds of PCR positivity (OR=2.76, 95% CI=1.61-5.03). Model 4, which combined risk assessment and the presence of symptoms, had the best fit compared to other models with an AIC of 1066.9 and Tjur’s R2 of 0.025. IPW models revealed similar results, as summarized in Supplementary Table 2.
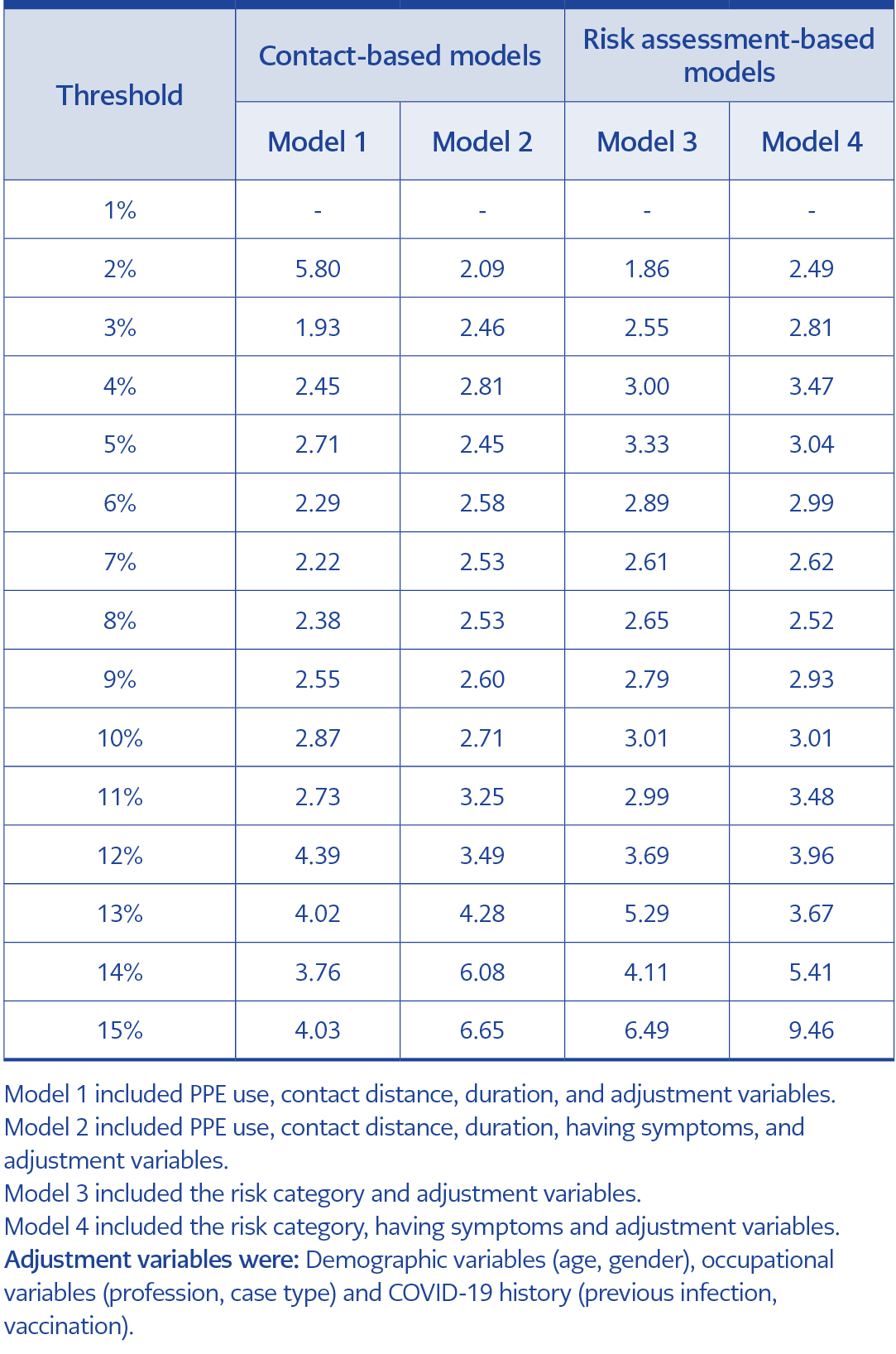
Table 5. The diagnostic odds ratios of the contact-based or risk assessment-based model thresholds range from 1% to 15%.
Table 5 shows the DOR calculated for four models, with varied thresholds ranging from 1% to 15%. At the minimum threshold (2%), Model 1 had the highest DOR of 5.80, and at the maximum threshold (15%), Model 4 had the highest DOR of 9.46.
Discussion
This study evaluated factors associated with developing RT-PCR positivity among exposed HCWs by analyzing one of the largest single-center contact tracing and exposure risk data from the COVID-19 pandemic. Our findings indicate that exposure risk assessment variables (i.e., use of PPE, contact distance and duration) significantly predict the development of infection, and whether the contact has symptoms or not improves this prediction.
Rigorous contact tracing can limit disease spread but may not be feasible once a substantial surge of new cases is reported daily (18). Managing a large number of in-hospital contacts requires significant human resources, timely communication, meticulous record-keeping, data management, and coordination efforts. As the full quarantine of exposed workers may be unrealistic, international organizations suggested exposure risk algorithms. Building upon the institutionally modified algorithms, our study found that a lack of PPE use and ≥15 minutes of contact duration increased the risk of RT-PCR positivity in contact-based models, but no association was found for contact distance.
Our findings are consistent with at least four meta-analyses (19-22), which also concluded that masks effectively prevent infection in HCWs. The data used in the studies was inherently based on one infector-infectee pair’s contact details. When there was widespread community transmission, the true protective effect of masking could be limited as prolonged mask use is difficult to adhere to, causing discomfort and breathing difficulties (23, 24). For this reason, a more strategic use of masks could be considered, making it stricter in high-risk areas such as those dealing with immunosuppressed patients or ICUs while adopting a more relaxed approach in other areas.
Contrary to a meta-analysis conducted during the early stages of the pandemic (25), this study found that contact distance had no significant effect on infection. Theoretically, social distancing reduces the exposure of virus-containing droplets/aerosols from one person to another as it limits the frequency of people sharing the same space. However, accurately assessing the contact distance proves challenging during the risk assessment as it may fluctuate throughout the contact period. Most existing literature focuses on country-level measures and outcomes, leaving a gap to explore person-to-person transmission dynamics (26-28).
The duration of contact is essential for risk assessment (9-12). We found that ≥15 minutes of contact increased the transmission risk. Integrating systematic pauses or breaks in prolonged healthcare activities could serve as a strategic intervention to limit direct contact and reduce transmission risk among HCWs. Controversially, for the workplace contacts of HCWs, Murugesan et al. did not identify a significant relationship between contact duration and symptom onset after exposure (29). Limiting contact duration during patient care is not always feasible in hospital settings, particularly in emergency care and surgeries. In such cases, controlling other measures, such as using masks and ventilation, should be critical to minimize transmission from a definite COVID-19 case.
Our risk assessment procedure indicated a connection between the presence of symptoms and transmission, which aligns with the findings of Mark et al. (5). Despite its significance, symptom status is not directly a part of risk assessment procedures (7, 8, 16). Incorporating symptom-based approaches into risk assessment models can pose challenges. Lack of confirming the probable COVID-19 case due to the not-perfect sensitivity of RT-PCR may lead to operational confusion of exposed HCW management. Additionally, the subjective nature of symptom reporting introduces prolonged sick leaves.
Similar to the findings of Durmaz et al. and Kalem et al., this study identified a 4.2% PCR positivity (144/3389) among contacts, with reported symptoms at 24.3% during interviews, notably higher (10, 11). During the pandemic, protective measures like prolonged mask-wearing may have led to symptoms (headaches, fatigue) that could be mistaken for COVID-19. Healthcare workers may have meticulously examined these symptoms, which could blend with genuine COVID-19 indicators. Additionally, PCR test sensitivity and sampling issues might have obstructed complete diagnostic accuracy. Despite our healthcare staff not being restricted by testing limitations, early national policies that curtailed PCR testing for asymptomatic cases may have impacted symptom reports. Furthermore, the presence of a group in the study that did not undergo testing could have also influenced the PCR positivity rate.
One of the important practical applications of the study lies in its ability to empower decision-makers with infection probabilities and discrimination power of the models based on real-world data. Through a user-friendly web-embedded platform, users can input relevant variables, enabling the models to generate real-time predictions for newly emerged respiratory virus infections that could require a response on a global scale. By utilizing this, the institutions can efficiently save time and personnel resources. We suggest lower probability thresholds and models with higher DORs for departments where infection-prone patients are admitted, such as hematology and oncology units, to minimize the HCW to patient transmission.
This study had several limitations. Initially, it was subject to recall bias, as contact details were based on personal statements. Subsequently, ascertainment bias could have arisen from unequal PCR testing probabilities among contact risk categories. We attempted to address selection bias by rebuilding inverse probability-weighted models. Furthermore, the single-center nature of the study may limit its generalizability to other hospital settings and other countries. To establish the utility of the models, external validation using datasets from different institutions is necessary. Due to the limited availability of variant analysis kits in our institution, comprehensive data on variants were lacking, potentially causing overlooked differences attributed to various variants. Lastly, this study may not have fully differentiated between workplace infections and community-based acquisitions. Additionally, it may not always be clear whether a contact was already positive prior to the interaction, independent of the exposure event. However, the policy within our institution allows for rapid PCR screening of symptomatic employees, which suggests that such cases are likely rare.
In conclusion, in this tertiary hospital study, the inclusion of being symptomatic made an improvement on the contact-based and risk assessment-based models. Based on these data, institutions with sufficient resources are encouraged to incorporate symptom inquiries into their risk assessment protocols in response to newly emerged respiratory virus infections that may seriously affect public health. Meanwhile, at minimum, institutions lacking the capacity for extensive contact tracing are recommended to track symptomatic exposed HCWs for outbreak control. Aiming for a zero-infection policy is extremely challenging and even unrealistic in the long term; hospitals should focus their mitigation efforts on evidence-based strategies, consequently enhancing both patient and HCW safety.