Introduction
Acinetobacter baumannii is one of the most pathogenic nosocomial infection agents because of its extreme resistance to almost all known antibiotics and host immune responses (1). The emergence of colistin-resistance in A. baumannii has been reported throughout the world (2, 3).
Biofilm formation ability enhances virulence of A. baumannii by increasing survival of cells in unfavourable environmental conditions, such as underexposure of disinfectants, antibiotics or attack of immune cells (4, 5). Antibiotic-resistant phenotypes strongly influence biofilm forming capacity of A. baumannii (6). The expression of biofilm-associated virulence genes and biofilm thickness in MDR (multidrug-resistant) strain was found to be higher than drug-sensitive strain (7).
A. baumannii acquires colistin resistance by rapid induction of resistance mechanisms in the presence of colistin (8). Simultaneously, bacteria display an adaptation to colistin exposure with significant changes in transcriptome profile and cell membrane structure (9). Biofilm-related outer membrane lipoprotein (pgaB) was found to be upregulated in colistin resistant strains (10). However, another study showed that mutations involved in colistin resistance phenotype downregulate the expression of biofilm-associated genes of colistin-resistant isolates (11).
The understanding of bacterial pathogenesis has lead to the development of many potential strategies and novel drugs to treat MDR bacteria. Anti-virulence treatment is one of the promising therapy approaches (12). The aim of this study is to explore the alterations in biofilm formation capacity of A. baumannii during adaptation to colistin resistance under colistin stress.
Materials and Methods
Study population and bacterial strains
We included 18 patients with isolation of A. baumannii between October 2014 and September 2018 from different centres in Turkey. The gender, age, source, carbapenem resistance, carbapenemase type, colistin exposure, and colistin resistance data were recorded. For all patients one representative A. baumannii (nine colistin resistant and nine colistin susceptible) isolate was included. Additionally, we selected two patients that developed colistin resistant A. baumannii infection during their hospital stay. The colistin susceptible and resistant pairs of A. baumannii isolated during the patients’ hospital course were studied.
Antibiotic susceptibility testing
Colistin minimum inhibitory concentrations (MICs) were determined by broth microdilution method. The isolates were grown on Tryptic Soy Agar (TSA) (Becton, Dickinson, U.S.) overnight. Then, the turbidity of each isolate was adjusted to 0.5 MacFarland by cation-adjusted Mueller Hinton broth (MH) using nephelometer (Becton, Dickinson, U.S.). Serial dilution was performed with final concentrations between 256 μg/mL and 0.25 mg/L. The samples were incubated at 37°C overnight and MICs were determined by measuring absorbance values at 540 nm in addition to reading by the naked eye. Escherichia coli ATCC 25922 standard strain was used as a reference, and resistance breakpoint was set as >2 μg/ mL based on Clinical and Laboratory Standards Institute (CLSI) guideline (13).
Assessment of biofilm formation
Biofilm production was measured by Crystal Violet Assay. Thus, all isolates were grown on TSA overnight. Then, a single colony was inoculated into 5 mL of Tryptic Soy Broth (TSB) and incubated at 37°C on a shaker (125 rpm) until turbidity reached 10-13 MacFarland. The cultures were diluted to 1:50 using TSB containing 0.1% glucose. 100 µL of diluted cultures in 96-well plate was incubated at 37°C for 24 h. After removal of non-adherent bacteria, adherent bacteria were fixed by incubation at 60°C for 40 min and stained with 125 µL of crystal violet. Bound crystal violet was dissolved by 95% ethanol. Optic Density (OD) values of wells were measured at 540 nm. The isolates with ODs between 0.12> and £0.5 were considered weak, >0.5 were strong biofilm producers (14).
For imaging of biofilms, bacterial cells were fixed with 3.5% formaldehyde solution overnight. The fixed cells were stained using the Live/Dead Backlight viability kit with following the manufacturer’s protocol. Samples were examined with Leica DMI8 laser scanning confocal microscope (15, 16).
In vitro adaptation study
For in vitro adaptation, four colistin susceptible strains (K399, K408, K411 and K412) of one of the patients with both colistin resistant and susceptible A. baumannii isolation were selected. The selected susceptible isolates were sub-cultured onto Mueller Hinton agar (MHA) containing 1 µg/mL colistin (Sigma) by 40 serial passages. Biofilm production and colistin MICs of each generation were determined.
Genotyping of isolates
MLST was performed by amplifying seven housekeeping genes, namely, cpn60, fusA, gltA, pyrG, recA, rplB, and rpoB according to the protocol developed by University of Oxford on A. baumannii MLST website (https://pubmlst.org/abaumannii/). Allelic profiles and sequence types (STs) were determined using Applied Math Bionumerics V7.6 software.
Results
Among 18 patients, seven of them were male. All of the patients with colistin resistant A. baumannii isolation received colistin therapy. Twelve of the isolates (67%) were obtained from blood cultures. All isolates were carbapenem resistant and OXA-23 producers (Table 1).
Data of the two patients that develop colistin resistant A. baumannii infection during hospital stay was presented in Table 2. Both patients received colistin therapy, and the patient 2 died after four days of A. baumannii isolation. All isolates belonged to global ST2 clone.
Biofilm formation of A. baumannii isolates
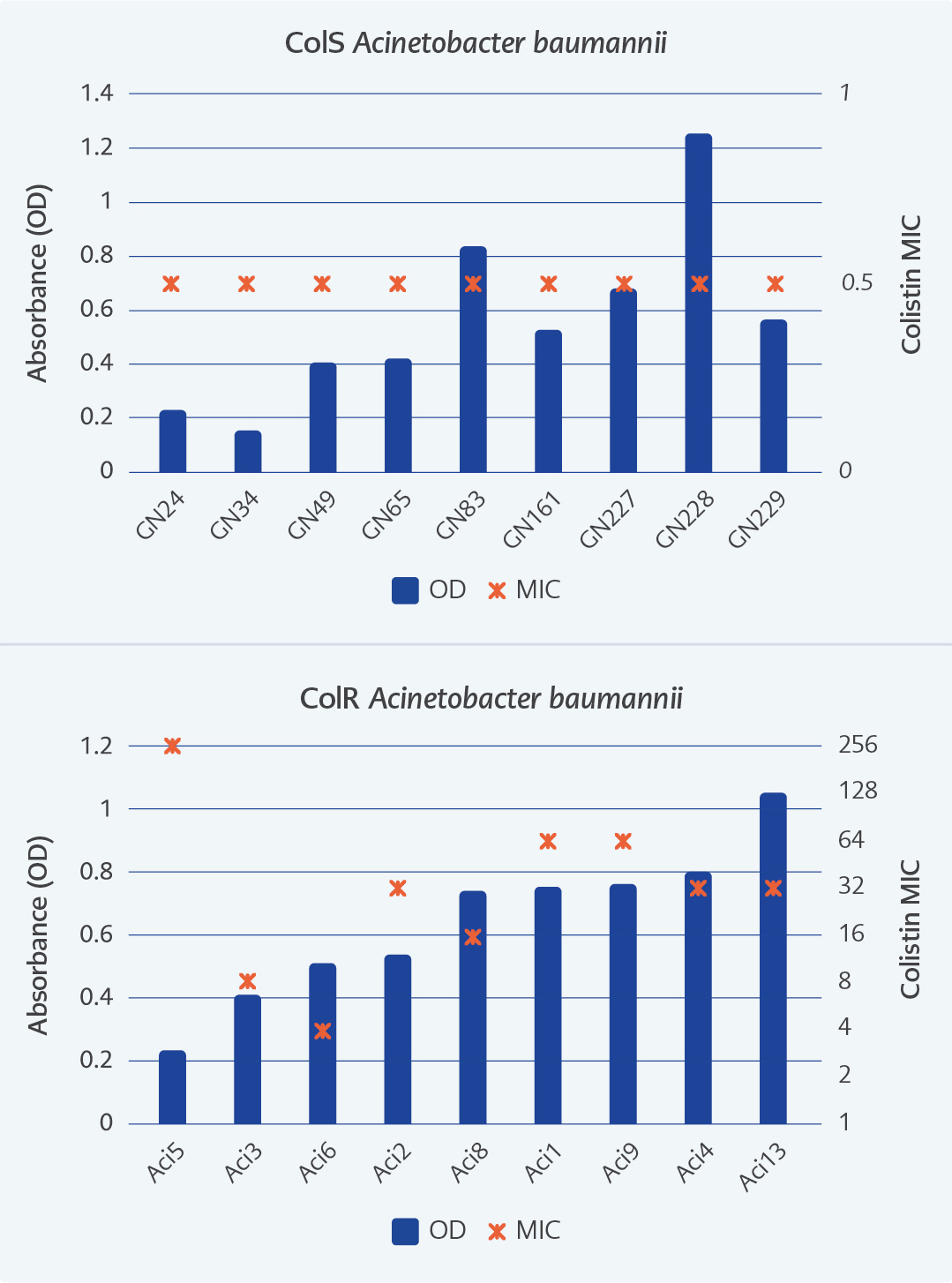
Figure 1. The biofilm and MIC levels of ColS and ColR A. baumannii isolates. OD: Optic Density; MIC: Minimum inhibitory concentration.
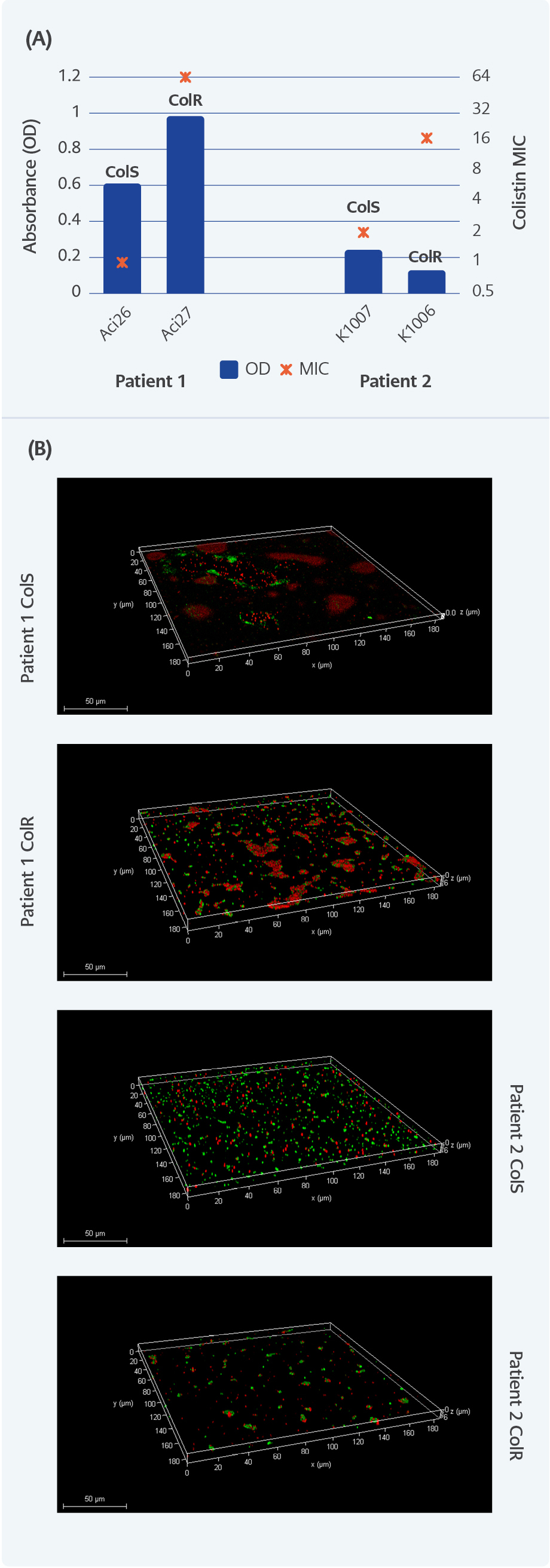
Figure 2. The biofilm and MIC levels of ColS-ColR A. baumannii pairs from two selected patients. Crystal violet assay and MICs (A); confocal images of biofilms (B). In confocal images, red color indicates dead, green color indicates live cells.
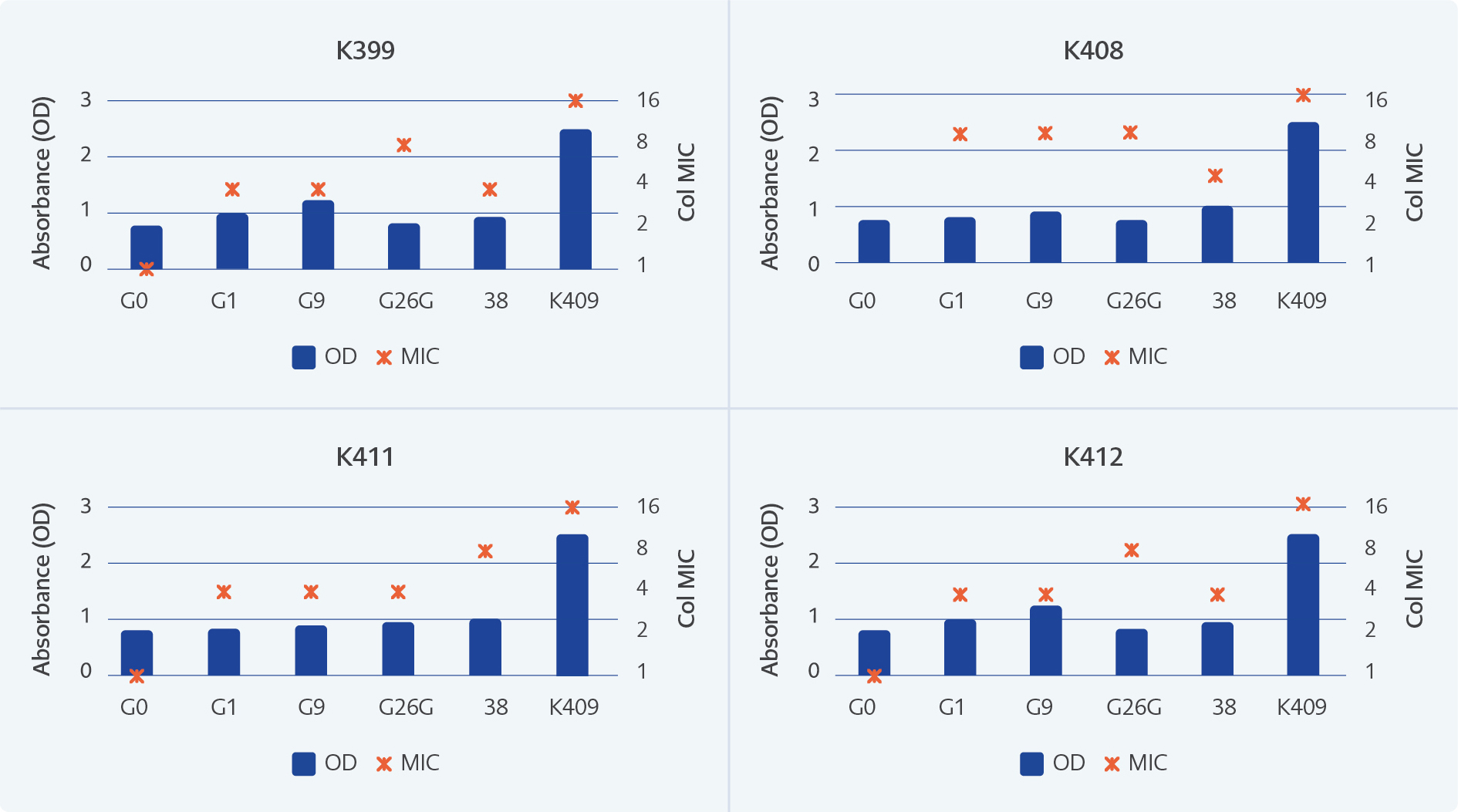
Figure 3. Biofilm production and colistin MICs of selected generations during in vitro adaptation to colistin exposure. OD: Optic Density; MIC: Minimum inhibitory concentration. K399, K408, K411 and K412 are the numbers of each isolates.
The biofilm production of ColR and ColS isolates were shown in Figure 1. Among all A. baumannii, 54% (5/9) of ColS isolates and 78% of ColR (7/9) were found to be strong biofilm producers. The median of OD values for ColS isolates was 0.53 (0.23-1.24), median for ColR isolates was 0.73 (0.12-1.05). In seven of the nine ColR isolates, the elevated colistin MICs were found to be accompanied to high levels of biofilms.
The biofilms and MICVs of A. baumannii from two selected patients were shown in Figure 2. In patient 1, biofilm production of ColR isolate was higher than ColS original strain; however, a slight decrease was observed in biofilm production of ColR strain than ColS counterpart in patient 2 (Figure 2A). Also, microscope images showed that biofilm productivity of ColR strain was stronger than ColS in patient 1, but there was a decrease in biofilm formation of ColR isolate compared to ColS strain in patient 2. These results were consistent with the crystal violet results.
Biofilm production of A. baumannii generations during in vitro adaptation to colistin exposure
The biofilm production and colistin MICs of selected generations of four colistin susceptible isolates (K399, K408, K411, and K412) and ColR isolate (K409) from patient 1 were shown in Figure 3. The Colistin MICs were increased to resistance breakpoint (2 mg/mL) at the first generation.
The results showed no considerable difference in biofilm formation between the generations. The K409 ColR clinical isolate had 2.6-3.4 fold stronger biofilm production capacity than laboratory induced colistin resistant generations.
Discussion
The emergence of colistin resistance in A. baumannii has increased the need for the development of new therapeutic approaches. Inhibition of virulence factors is becoming one of the most popular strategies for the treatment of infections (12, 17). Biofilm formation of A. baumannii is a significant virulence determinant that because of the strong interaction of bacteria with host cells (7).
Here we presented 18 cases with an isolation of A. baumannii from different specimens. 17 % of total isolates and 33 % of ColR isolates were from respiratory specimens. The biofilm production rate of all isolates was 67%. In 2008, a multicentered cohort study showed 63% biofilm formation among A. baumannii isolated from various sources. They also reported that respiratory isolation was associated with non-biofilm production (18). Controversially, in another study, high-level biofilm formation was observed in respiratory specimens of 61 patients in 2013 (19).
In this study, we detected strong biofilm production (OD>0.5) in 78% of ColR, and in 54% of ColS A. baumannii isolates. Recent studies reported a significant association between high biofilm formation capacity and multidrug-resistant profile of A. baumannii (20). A multi-centric hospital based study showed that more than 90% of the biofilm producer A. baumannii isolates were multidrug-resistant (6). Additionally, MDR and biofilm producer A. baumannii strains were reported from outbreaks in hospitals especially in intensive care units (21, 22). However, there are controversial reports about the colistin resistance with biofilm production. Farshadzadeh et al. (11) found that the biofilm-forming ability of ColR A. baumannii was not significantly different from their ColS counterparts. They claimed that biofilm formation capacity could be related to the change in growth rate. In two different studies, Pournaras and Dafopoulou revealed that the acquisition of colistin resistance via a single pmrB mutation was associated with an impaired biofilm formation capacity because of the decrease in growth rate (23, 24). Another important finding of our study was the association of high colistin MICs with high biofilm values among ColR A. baumannii. A very recent study reported overexpression of biofilm-associated genes underexposure of colistin (25). In our study, all patients received colistin therapy before isolation of ColR strains.
In this study, we also examined the alterations in biofilm ability of A. baumannii during the in vitro adaptation period to colistin resistance and compared the in vivo and in vitro results. The ColR (K409) isolate was detected on the 25th day of the colistin therapy. This isolate was found to be a strong biofilm producer revealed 2.6-3.4 times more biofilm levels than ColS first pair. When we mimicked in vivo colistin use in the laboratory, the exposure of sub-MIC concentration of colistin (1mg/L) did not alter biofilm production of the generations. Even the 25th generation that corresponds to the duration of colistin therapy at the isolation day of clinical ColR A. baumannii did not show a difference than ColS first pair. These results suggested that colistin exposure is not the only factor that affects the in vivo biofilm formation ability of A. baumannii under colistin stress. Other contributors might have a role in biofilm production of bacteria during the development of colistin resistance. Similarly, a study published in 2018 declared that colistin exposure did not have an effect on the biofilm-forming capacity of laboratory-induced A. baumannii (26). Besides, recent in vitro studies reported the biological cost of adaptation to colistin resistance in A. baumannii. The loss of LPS leads fitness cost and low biofilm formation capacity so consequently reduces virulence (11, 27, 28).
In conclusion, the biofilm forming capacity of ColR A. baumannii isolates is higher than ColS A. baumannii. On the other hand, we did not find a difference in biofilm levels of laboratory-induced colistin resistant generations. We suggest that A. baumannii may develop adaptation mechanisms to constitute colistin-resistance in the presence of host-dependent factors and environmental stress conditions in order to gain stronger biofilm production capacity to enhance virulence.